Mohd. Zahid Ansari , Jitendra Jangir , Lokesh Verma , Vikram Singh
PDPM-Indian Institute of Information Technology, Design and Manufacturing (IIITDM) Jabalpur, Khamaria, Jabalpur, India
Correspondence to: Mohd. Zahid Ansari , PDPM-Indian Institute of Information Technology, Design and Manufacturing (IIITDM) Jabalpur, Khamaria, Jabalpur, India.
Email: |  |
Copyright © 2015 Scientific & Academic Publishing. All Rights Reserved.
Abstract
Sandwich composites are an important class of engineering materials that provide very strong yet light weight, high impact energy absorbing and high temperature operating ability. This paper studies the compressive behaviour of honeycomb sandwich composites made of hexagonal aluminium core and acrylic polymer skin using experimental and numerical methods. Prepared sandwich samples were fabricated and tested for maximum compressive strength. In addition, finite element analysis was conducted to characterize the deformation, stress and thermal insulation properties of another aluminium alloy sandwich in out-of-plane loading. The sandwich plates were subjected to compressive load, whereas, the beams were subject to bending load. Thermal insulation study on sandwich with acrylic skin was also conducted. Experimental results showed that compressive strength of the sandwich with acrylic skin can support a distributed maximum load of 4 MPa. In addition, the thermal insulation property of this sandwich is found to be better than a wood plate.
Keywords:
Honeycomb, Acrylic polymer, Cellular solid, Sandwich composite, Finite element analysis (FEA)
Cite this paper: Mohd. Zahid Ansari , Jitendra Jangir , Lokesh Verma , Vikram Singh , Compressive Behaviour of Polymer/Honeycomb Sandwich Composites, American Journal of Materials Science, Vol. 5 No. 3C, 2015, pp. 112-115. doi: 10.5923/c.materials.201502.23.
1. Introduction
Honeycomb is basically a cellular structure where highly ordered thick cells are separated by thin walls of material to form a planar structure. Normally, the shapes of the cells are hexagonal, but square and rectangular shapes are also available. The structure was originally observed in natural honey beehives. Honeycomb materials belong to a special class of engineering materials called cellular solids. These solids have a highly porous structure with many voids and bobbles inside. The porosity is normally more than ninety percent by volume. Honeycombs can be made of almost any material depending on the application. The materials can be metal, polymer, ceramic and glasses. Al honeycombs are most commonly used as the core material in sandwich composites to impart lightweight and high strength properties. As such, they are used in automobiles, aerospace, naval and other lightweight yet strong load supporting application. The honeycombs have a planar structure and are normally designed to support out-of-plane compressive or bending loads. The loading can be purely compressive or bending load wherein the compressive as well as tensile loads occur simultaneously. Sandwich composites provide a number of structural advantages over conventional engineering materials such as high strength-to-weight ratio, high impact load resistance and high thermal insulation properties [1, 2]. Therefore, sandwich structures are commonly used in automobiles, aircraft, aerospace vehicles, ship structure, medical implants and safety equipments. Sandwich is basically a type of thick composite where two thin and high strength skin sheets are bonded adhesively to a thick and low strength core in between. Sandwich composites have very good out-of-plane loading strength. The skin of a sandwich supports normal tensile and compressive loads, whereas, the core supports mainly the shear load [1]. By varying the core and skin materials and designs, efficient engineering structures and designs can be achieved. The skin of the sandwich can be thin sheet of metals, alloys or another composite material like carbon-fibre reinforced plastic and glass-fibre reinforced plastic [3, 4]. Similarly, the core material can be wood or a cellular solid material like honeycomb and foam. This study uses thin acrylic sheet as skin and thick hexagonal aluminium honeycomb as the core of the sandwich in experiments. The samples were fabricated and tested for maximum through-thickness compressive load supporting ability [5]. Commercial finite element analysis software ANSYS Workbench was used to study the compressive, bending and thermal characteristics of sandwich plates and beams made of acrylic and aluminium skin and aluminium honeycomb core.
2. Experiments and Simulation
Figure 1 shows the sandwich sample before and after the compression test. The skin was made of 5 mm thick acrylic polymer sheet and the core was made of low density aluminium honeycomb. The height of the honeycomb was 25 mm. The cell size was 6 mm and the wall thickness was 0.02 mm. The honeycomb samples were cut from the block using grinder cutter. The core was bonded adhesively to the skins using an epoxy adhesive resin. Five samples were tested for static compression using a universal testing machine. Typical sample size was 50×50×35 mm. 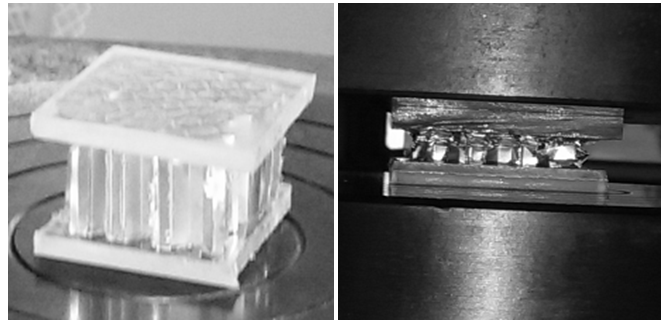 | Figure 1. Experimental honeycomb sandwich sample before (top) and after (bottom) the compressive tests |
In order to better understand and visualize the mechanical properties like compression, bending and thermal insulation of the honeycomb sandwich composites, a commercial finite element analysis software ANSYS Workbench was used to study the aluminium alloy honeycomb sandwich with same core and skin material in form of thick plate and beam. Figure 2 shows the computer-aided design (CAD) model of the sandwich. The design was made using CAD software SolidWorks and was imported into Workbench for finite element analysis. 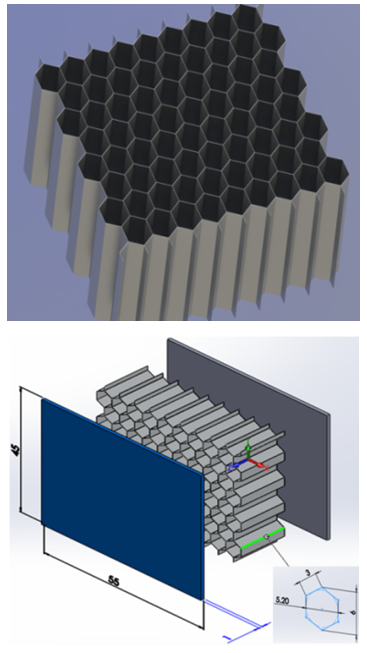 | Figure 2. CAD models of aluminium honeycomb and sandwich panel |
Figure 3 shows the finite element model of the plate and beam structures of the sandwich. The size of the sandwich plate was 55×45×17 mm, whereas the size of the sandwich beam was 210×40×17 mm. The core and skin thicknesses are 15 mm and 1 mm, respectively. The elastic modulus and Poisson's ration of the aluminium core and skin are 71 GPa and 0.33, respectively. And, the compressive yield strength of is 280 MPa. The sandwich plate was exposed to compressive and thermal loads, whereas, the beam was subject to uniformly distributed transverse bending load. 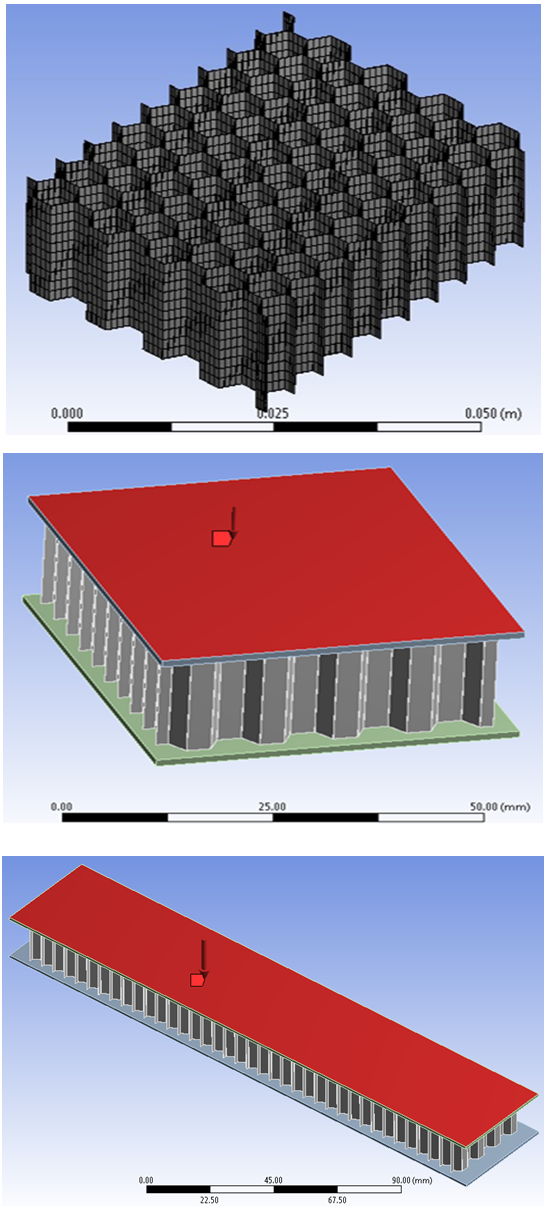 | Figure 3. Finite element models of sandwich plate and beam |
3. Results and Discussion
Figure 4 presents the average force-displacement characteristics of the honeycomb core under compression. The samples were compressed by about 10 mm, which corresponds to a strain of 40%. The annotation in figure 3 indicate the experimental data for the onset of material yielding and collapsing. The curve show a behaviour typical of cellular solid like foam and honeycomb material and has three distinct deformation zones, namely linear elastic, plastic plateau and densification. 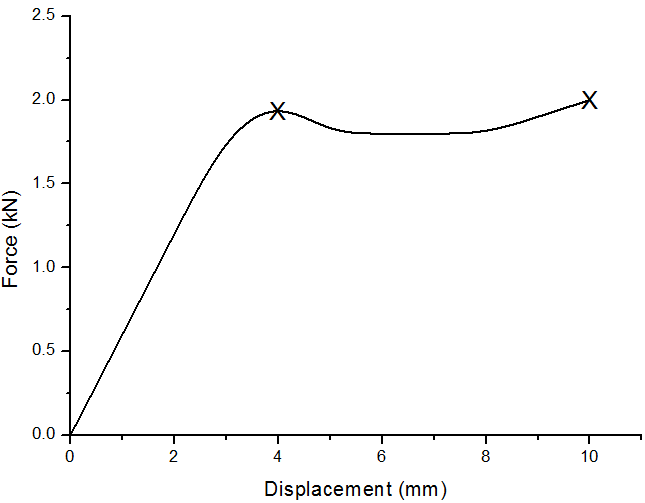 | Figure 4. Compressive force-displacement behaviour of sandwich samples |
In our experiments, we limited the deformations up to the plateau zone only. The linear elastic zone extends up to the strains of about 3 mm. This zone presents the deformation where the cell walls of the honeycomb are compressed axially. Once the walls start to buckle, the plateau zone starts wherein the material begins to yield and buckle under compression and the deformation curve becomes parallel. Figure 1 shows the example of collapse sandwich sample.Figure 5 shows the numerical results for deformation and stress of the aluminium alloy honeycomb sandwich with same core and skin material under a compressive load of 4 MPa. The average centre deformation is less than 1 mm. It suggests the high compressive strength of the sandwich. The stress distribution results show a uniform distribution of stress in the centre of the sandwich and the maximum stress is about 233 MPa. This value is less than the compressive yield stress of 280 MPa for the aluminium. Thus, by considering the factor of safety the sandwich plate showed in figure 3 can support a maximum load of 4 MPa, which corresponds to an out-of-plane distributed load of about 9.9 kN on sandwich plate of size 55×45 mm. It should be noted that this sandwich composite properties used in simulations may be different from the ones used in experiments because the material properties of the aluminium core provided by the vendor and used in the experiments are unknown. Simulations used the standard material properties of aluminium.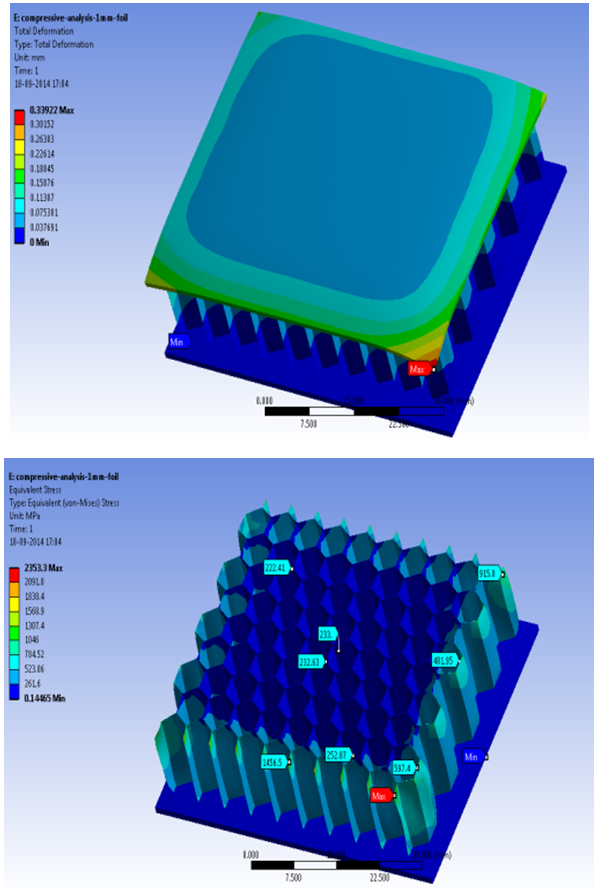 | Figure 5. FEA results for deformation (top) and maximum stress (below) for compressive load of 4 MPa in sandwich plate |
Figure 6 shows the numerical results for deformation and stress of the aluminium alloy honeycomb sandwich beam under compressive load of 500 kPa. The two ends of the beam are fully fixed. In this case, the maximum deformation of about 1.2 mm is occurring in the mid-span of the beam. The maximum stresses are occurring at the fixed ends of the beams because of the fully constrained boundary condition. The maximum stress value is about 1.6 GPa, which is far higher than the yield strength of aluminium at 280 MPa. However, since in practice the boundary conditions would not be as restrictive as in the numerical analysis, the maximum stress induced would be much lower. A compressive load of 0.5 MPa corresponds to an out-of-plane distributed load of about 4.3 kN on sandwich beam of size 210×40 mm.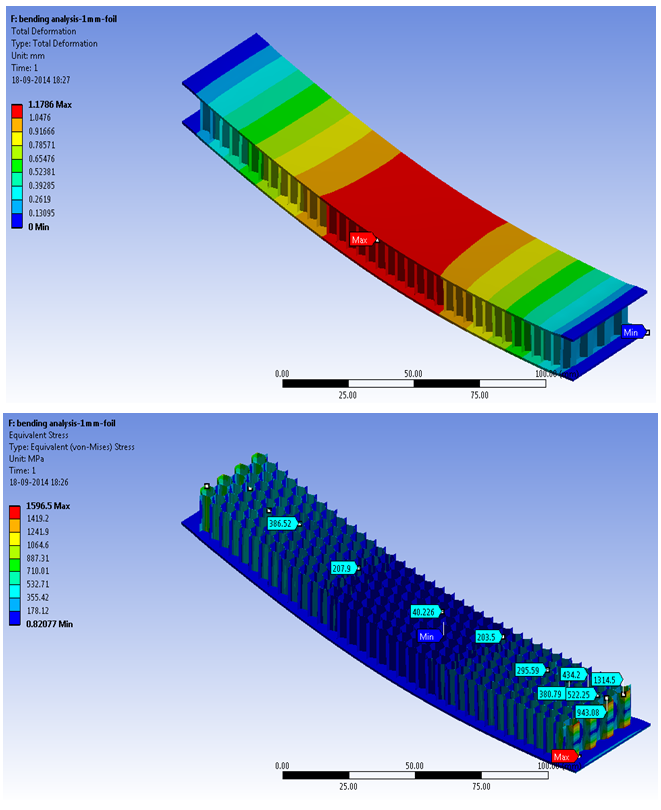 | Figure 6. FEA results for deformation (top) and maximum stress (below) for compressive load of 500 kPa in sandwich beam |
Figure 7 shows the numerical results for temperature distribution in the aluminium sandwich plate with acrylic skin and a solid wooden plate when used in thermal insulation application. One face of the plate is maintained at 55°C and the plate is exposed to ambient air with temperature 22°C and convective heat transfer coefficient 5 W/m2°C. The thermal conductivities of acrylic, aluminium and wood are assumed about 0.2, 237 and 0.05 W/m°C, respectively. It is obvious in the figure that the thermal insulation capability of honeycomb is higher than wood. Compared to creating a temperature gradient of about 25 °C in case of wood, the sandwich is creating a gradient of about 33°C. Thus, honeycomb is clearly a better choice for thermal insulation.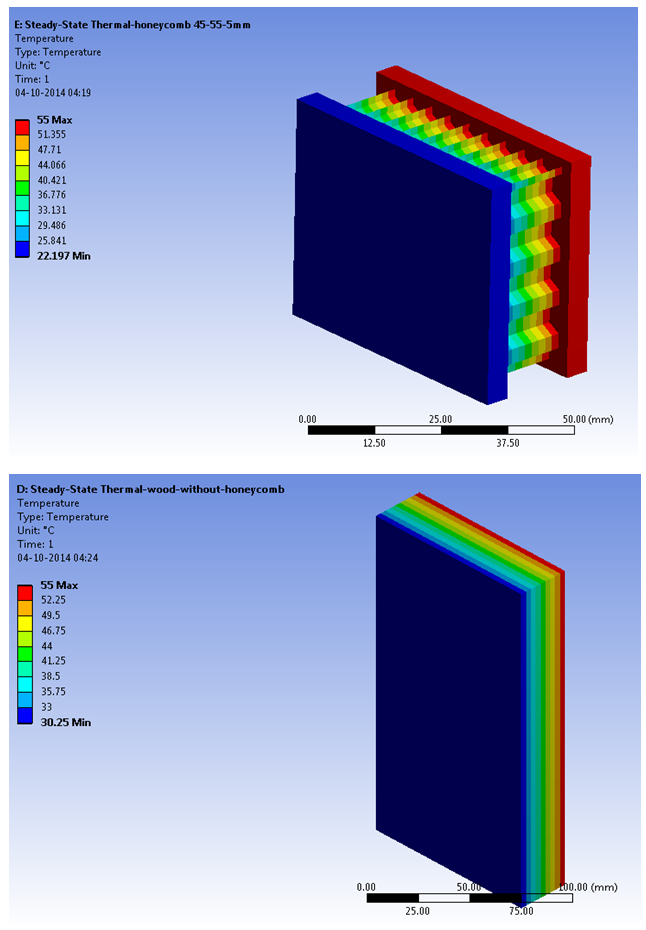 | Figure 7. FEA results for temperature distribution in sandwich (top) and wood (below) plates |
The sandwich composite plate and beam designs presented in this study can be used in structural application like doors and walls of a pre-fabricated structure and the thermal insulation structure which can also support load. In addition, the very high impact energy absorption ability of honeycomb sandwich structures can become very useful in applications where protection against collision and impact is required.
4. Conclusions
This study used experimental method to investigate the compressive strength of aluminium honeycomb sandwich composite under compressive load. In addition, finite element analysis was conducted on aluminium alloy honeycomb sandwich with same core and skin material to study the deflection and stress characteristics of sandwich plate and beam under out-of-plane loading. During compression test, the prepared sandwich samples showed the typical force-displacement behaviour of a honeycomb sandwich with linear and plateau zones. Results showed the sandwich plate samples can support a distributed compressive load up to 4 MPa. The samples failed by yielding and buckling at this load. Numerical results using finite element analysis on aluminium alloy skin and core sandwich plates showed a maximum deflection of 1 mm occurs for the load of 4 MPa and the plates can support this load without failure. Similarly, the bending test on this sandwich beam showed a deflection 1.2 mm at distributed load of 0.5 MPa, but the sandwich will start to yield due to very high local stresses in the honeycomb. Finally, the thermal insulation results showed that sandwich composite is providing an insulation which is about 8°C than wood. Thus, honeycomb is more suitable for thermal insulation.
ACKNOWLEDGEMENTS
This study was supported by Indian Institute of Information Technology, Design and Manufacturing-Jabalpur.
References
[1] | L. J. Gibson and M. F. Ashby, Cellular Solids: Structure and Properties. Cambridge University Press, 1999. |
[2] | S. Abrate, Impact on Composite Structures. Cambridge: Cambridge University Press, 1998. |
[3] | S.K. lee, "A study on penetration failure of CFRP/porous metal core sandwich composites", PhD Thesis, Inha University, South Korea ,2012. |
[4] | S. K. Lee, M. Z. Ansari, N. Wang, and C. Du Cho, “A Computational Study on Residual Strength of Sandwich Composite Panel,” in Advanced Materials Research, 2011, vol. 393–395, pp. 521–525. |
[5] | F. Coté, B. P. Russell, V. S. Deshpande, and N. A. Fleck, “The Through-Thickness Compressive Strength of a Composite Sandwich Panel With a Hierarchical Square Honeycomb Sandwich Core,” J. Appl. Mech., vol. 76, no. 6, p. 061004, Nov. 2009. |