Prashanth Kumar 1, Vijeth 1, Peter Fernandes 2, K. Raju 1
1Department of Mechanical Engineering, St. Joseph Engineering College, Mangaluru, India
2Department of Mechanical Engineering, Alva’s Institute of Engineering and Technology, Moodbidri, India
Correspondence to: K. Raju , Department of Mechanical Engineering, St. Joseph Engineering College, Mangaluru, India.
Email: |  |
Copyright © 2015 Scientific & Academic Publishing. All Rights Reserved.
Abstract
In the present study, bio-fuel prepared from the blends of cotton seed methyl ester (COME), sapindus mukorossi oil (SNO) and diesel has been tested on a CI engine at an injection pressure of 240 bar. Its performance and emission characteristics are studied and reported. It was found that the brake thermal efficiency of COME25SNO5 was higher than that of diesel by 3%. At the maximum load the brake specific fuel consumption of COME15SNO5 and COME20SNO5 blends are 2% higher than that of diesel and for COME20SNO5 blend, the brake specific fuel consumption is 10.5% higher than that of diesel. At full load the COME15SNO5 blend and neat diesel have the same percentage of CO emission. Smoke opacity of diesel is higher compared to the blended fuels.
Keywords:
Cotton seed oil methyl ester, CI engine, Emission, Sapindous mukorossi oil
Cite this paper: Prashanth Kumar , Vijeth , Peter Fernandes , K. Raju , A Study on Performance and Emission Characteristics of Cotton Seed Methyl Ester, Sapindous Mukorossi Seed Oil, and Diesel Blends on CI Engine, Energy and Power, Vol. 5 No. 1A, 2015, pp. 10-14. doi: 10.5923/c.ep.201501.03.
1. Introduction
The use of vegetable oils and its derivatives in diesel engine has begun about 115 years back. The inventor of the diesel engine, Rudolf Diesel reportedly used peanut oil as fuel for demonstration purposes in 1900. Biodiesel is an alternative to diesel fuel which can be produced from different sources such as animal fats or vegetable oils. It has many advantages over diesel fuel; as it is biodegradable, renewable and non-toxic. The properties of biodiesel may vary significantly depending on their chemical compositions and fatty acid composition, which give obvious effect on performance and emissions. Cottonseed oil is a vegetable oil extracted from the seeds of the cotton plant after the cotton lint has been removed.Hseyin Aydin et al. [1] conducted performance and emission analysis of cottonseed oil and methyl ester in a diesel engine with blends of B5, B20, B50 and B100 and reported there is a decrease in torque with an increase in blend ratio due to higher viscosity and lower heating value. At full load, BSFC with B20 was lower than diesel at low speed due to fuel based oxygen and higher cetane number, that lead to complete combustion. CO emission decreased with biodiesel and NOx emission decreased except for B5.Md Nurun Nabi et al. [2] conducted a study on cotton seed oil and its effect on engine performance and exhaust emissions with blends B10, B20, B30, B40 and B50 and reported that CO, PM emission and smoke levels were less than that of diesel due to oxygen content and low aromatics. Higher NOx emission when compared to diesel due to oxygen content. Thermal efficiency of biodiesel was slightly lower, due to lower heating value.Tizane Daho et al. [3] conducted a study to find the Influence of engine load and fuel droplet size on performance of a CI engine fueled with cotton seed oil and its blends with diesel fuel, CSO 20, 40, 60, 80 and 100. He reported that the thermal efficiency was better for B20 and unfavorable fuel droplet size distribution does not affect thermal efficiency. BSFC increases with the percentage of CSO and premixed combustion is less represented than diffusive phase. Large droplets in the spray cause spray deposits. R. D Misra et al. [4] conducted performance, emission and combustion evaluation of soapnut oil and diesel blends in a compression ignition engine and reported that at lower blends of SNO and diesel, power output and fuel consumption were same. At SNO10 brake thermal efficiency was found to be same as diesel. At higher blends, emission of HC and CO are higher and SNO10 and SNO20 resulted in 11% and 2% reduction in NOx respectively. SNO10, SNO20 and SNO30 have less smoke opacity.Chang Sik Lee et al. [5] conducted study on the fuel injection and atomization characteristics of soya bean oil methyl ester and reported that, as injection pressure increased injection delay decreased due to the increase of fuel momentum by injection pressure. Real injection duration increased about 30%. The SMD (sauter mean diameter) of biodiesel fuel decreases along the spray axis, interaction between the spray and the ambient gas affects the fuel atomization.Cenk Sayin et al. [6] reported the performance of single cylinder diesel engine by using blends of canola methyl ester with diesel and also by varying the injection pressure. Brake thermal efficiency was found lower using canola oil methyl ester compared to that of neat diesel. As the injection pressure increased from set pressure better results were found for brake thermal efficiency for canola oil methyl ester. Ismet Çelikten et al. [7] conducted a study on performance of 4 cylinder engine for three different injection pressures such as 250, 300 and 350 bar using diesel, rapeseed oil methyl ester and soybean oil methyl ester separately and reported that there is a reduction in engine torque, power, percentage of smoke and CO. NOx emission was found to be increased as the injection pressure increased for biodiesel. Soybean oil methyl ester had low torque and power as the injection pressure increased when compared to diesel and rapeseed oil methyl ester. Smoke and CO emission were less. NOx emissions were found higher when compared to other two fuels. Rapeseed oil methyl ester had lower power and torque when compared to that of neat diesel, but relatively higher than soybean oil methyl ester at all injection pressures. Smoke and CO emissions were relatively higher than soybean oil methyl ester but lower than diesel oil at all injection pressure. NOx emissions were lower than soybean oil methyl ester but higher than diesel at all injection pressures. Avinash Agarwal et al. [8] reported that there is an increase in thermal efficiency with increasing fuel injection pressure from 180 to 200 bars. Godiganur et al. [9] used 4 kinds of biodiesel and their blends for studying the performance and emission characteristics on C.I engine and reported that the increasing proportion of biodiesel in the blends causes increased NOx emissions. Rahaman et al. [10] reported that brake thermal efficiency of CI engine increased when fuelled with karanja methyl ester blended with diesel.
2. Experimental Details
The engine used in this experiment was a single cylinder, water-cooled, 4-stroke; DI diesel engine coupled with a dynamometer. The specifications of the engine are shown in Table 1. Fuel injection pressure is increased from the standard setting pressure of 180 to 240 bar by adjusting the spring pressure of the fuel injector. All experiments are conducted at standard temperature and pressure. The engine speed is measured directly from the tachometer attached with the dynamometer. The engine exhaust emissions HC, CO and NOx are measured with a portable digital gas analyzer (IMR 1400) and smoke opacity are measured with AVL 437C smoke meter. Three trials are conducted for each test and average of the results is presented in this report.Table 1. Specification of the engine 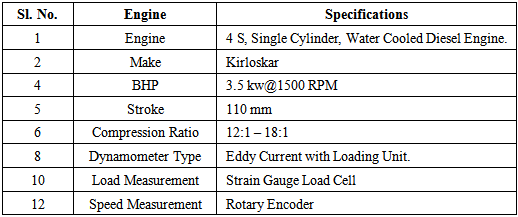 |
| |
|
2.1. Extraction of Sapindous Mukorossi Seed Oil by Solvent Extraction
Sapindous Mukorossi seeds are separated from the nuts; kernel of the seeds are removed and dried under sunlight for three days for removing the moisture content. Dry kernel is then ground into powder form, this powder is wrapped in a filter paper and placed on soxhlet apparatus.Approximately 1000 ml of hexane is filled in a RB Flask (Round Bottom Flask used for extraction was 3000 ml capacity) and is placed on the heating mantle. Above RB flask, the soxhlet apparatus is assembled that is filled with the hexane up to the bulb level. The condenser is then placed above the soxhlet apparatus, water passes through the inlet of the condenser. Then the round bottom flask containing hexane is heated to 50°C using a heating mantle. Hexane which gets evaporated will be passed through a bypass tube in soxhlet apparatus and then they will condense on the top with the help of a condenser. Condensed hexane will be collected in the soxhlet apparatus; hexane will pull out the oil.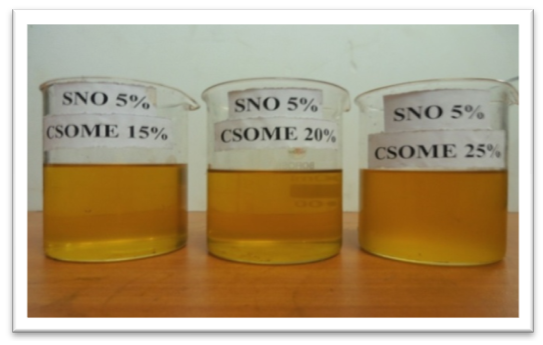 | Figure 1. Different blends of cottonseed methyl ester, sapindous mukorossi seed oil and diesel |
2.2. Transesterification of Cotton Seed Oil
Transesterification is one of the methods to reduce the viscosity of the vegetable oils. Cottonseed oil (1000 ml) is heated to 65°C in three neck flat bottom flask. Uniform heating is done using a magnetic stirrer with the speed of 600-950 rpm. 300 ml of methanol is taken in a separate beaker, 6.5 grams of sodium hydroxide flakes are added into it. Sodium hydroxide flakes are stirred to dissolve in methanol using a glass rod. The mixture is then added into three neck flat bottom flask that consists of crude cotton seed oil at 65°C, the reaction is carried out at 60°C for 2 hours.
2.3. Properties of Neat Diesel Fuel and Biodiesel Mixtures
Performance of CI engine greatly depends upon the properties of fuel among which viscosity, density, cetane number, volatility, and calorific value are important. The three blends with 15% COME and 5% SNO, 20%COME and 5% SNO and 25% COME and 5% SNO are prepared on volume basis. Properties of diesel and the three blends COME15SNO5, COME20SNO5, and COME25SNO5 are determined as per ASTM standard and results are tabulated in Table 2.Table 2. Different properties of diesel COME and SNO blends 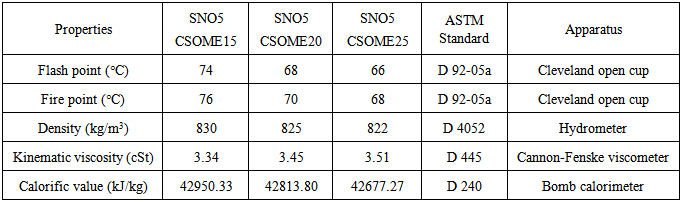 |
| |
|
3. Results and Discussion
Effect of load and blend on engine performance and emission characteristics of cotton seed methyl ester and sapindous mukorossi oil and diesel blends with pure diesel were investigated in the test engine. Experiments were conducted at 2, 4, 8 and 12 kg load.
3.1. Brake Thermal Efficiency (BTE)
BTE and BSFC are the performance parameters of the engine. BTE of CI engine increases with load for all the fuels used in the test. The effects of blends on BTE for different loads for engine are shown in the Figure 2. Generally BTE of the engine with biodiesel and its blends is less than diesel. This is due to reduced atomization, vaporization and combustion. It is found that increasing the injection pressure increases the atomization and increases the conversion efficiency. It is clear from Figure 1 that at maximum applied load BTE of COME25SNO5 has 3% higher than diesel at 240 bar injection pressure. Jindal et al. (11) also reported similar results.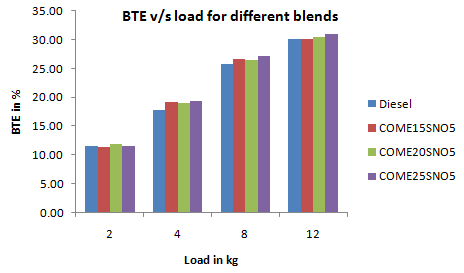 | Figure 2. Effect of blends and load on brake thermal efficiency |
3.2. Brake Specific Fuel Consumption (BSFC)
Figure 3 shows the effect of blends on BSFC for different loads for engine running on cottonseed methyl ester and sapindous mukorossi oil. BSFC decreases with increasing load for all the fuels used in the tests. Brake specific fuel consumption of all the blended fuels are higher than diesel. Since the calorific value of the blended fuel is less than diesel, more amount of blended fuel is consumed for developing the same power. At maximum load COME15SNO5 and COME20 SNO5 has 2% higher BSFC than diesel. For the other blend, marginal variations are found compared with diesel.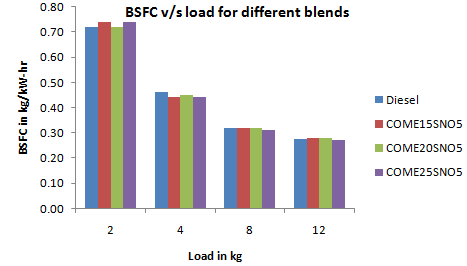 | Figure 3. Effect of blends and load on brake specific fuel consumption |
3.3. Emission Characteristics
3.3.1. Emission of NOx
Figure 4 shows the effect of blends and load on emission of NOX at 240 bar injection pressure. NOx increases with increase in load applied on the engine. Emission of NOx is the direct function of engine loading. This is expected because increase in load increases the combustion chamber temperature. The reasons for the formation of NOx are availability of oxygen at high temperature and retention time. It is clear from the Figure 4 that increase of percentage SNOCOME part in the blend increases the emission of NOx. SNO5COME25 at maximum applied load emits 10.5% higher NOx than diesel. At no load conditions emission of NOx for COME25SNO5 is 19.5% higher than diesel.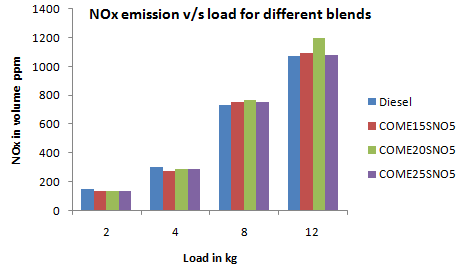 | Figure 4. Effect of blends and load on emission of Nox |
3.3.2. Emission of CO
Figure 5 shows the effect of blends on the emission of carbon monoxide for different loads. Emission of CO decreases with increasing load. CO is formed due to incomplete combustion of fuel. With increase in load combustion chamber temperature increases this leads to complete combustion of fuel and decreases CO emission. Biodiesel is an oxygenated fuel leads to complete combustion and reduces the emission of CO. Presence of vegetable oil content in the fuel increases the possibility of CO emission. It is clear from the Figure 4 that emission of CO is higher for all the higher blends compared to diesel and lower blends.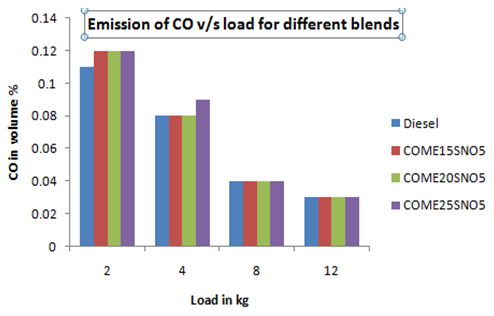 | Figure 5. Effect of load and blend on CO emission |
At low applied load blends of diesel has higher CO emission. For blended fuel CO emission is 7.7% lower than diesel. At higher applied load there is a marginal variation in the emission of diesel and other blended fuels.
3.3.3. Emission of UBHC
Figure 6 shows emission of UBHC for different blends at different applied loads. The exact reason for the formation of UBHC is not yet clear, but it may be due to partial combustion of fuel.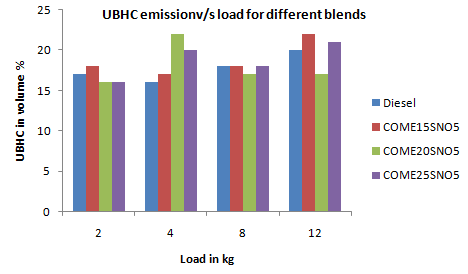 | Figure 6. Effect of load and blend on unburned hydrocarbon emissions |
At higher load emission of UBHC for all the blends are higher than at lower loads. It is 21 ppm for COME25 SNO5 and 20 ppm for diesel. At low load and high load operations, fuel with higher blends of biodiesel emits lower HC emission compared with diesel and lower biodiesel blends. At moderate load, emission of HC is higher for higher blends compared to diesel and lower blends of biodiesel.
3.3.4. Emission of Smoke Opacity
Smoke opacity increases with increase in load. Diesel has higher smoke opacity at lower load compared with the blends. Aromatic content in the fuel leads to increased smoke opacity. Aromatic content in the diesel are higher compared to the blends. Figure 7 shows effect of blends and load on smoke opacity. Diesel has highest smoke at low load compared to the other blends. At low load diesel has 55.7% higher smoke opacity than COME15SNO5. Smoke opacity increases with load.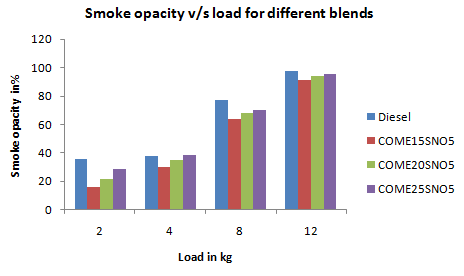 | Figure 7. Effect of load and blend on emission of smoke |
The difference in the smoke opacity decreases with increase in load. At maximum applied load COME15SNO5 has 6.5% lower smoke opacity than diesel.
4. Conclusions
The following conclusions may be drawn from the present study; 1. At 240 bar injection pressure it was observed that BTE with SNO5, CSOME25 as a fuel is 31% at maximum applied load compared with 30% with diesel as fuel. At low applied load it is 11.4% with diesel compared to 11.5% with COME25SNO5 as fuel. Blended fuels results slightly higher BTE at increased injection pressure when compared to neat diesel. 2. BSFC of the blended fuel is higher than diesel. At low load BSFC for diesel is 0.72 kg/kW-hr compared to 0.74 kg/kW- hr for blends of CSOME25SNO5. At Maximum applied load BSFC for diesel and blends are equal to 0.27kg/kW-hr. 3. CO emissions with diesel and blended diesel are low at maximum applied load. No much variations in CO emission at maximum applied load.4. UBHC emission in CSOME20SNO5 and CSOME25- SNO5 blends were lower at low load and high load operations. For moderate applied load, emission of UBHC is higher compared with diesel and lower blends of biodiesel. At low load, diesel has 55.5% higher compared to COME15SNO5. At high load 6.5% higher compared to COME15SNO5.5. Smoke opacity at maximum applied load with COME15SNO5 as fuel is 6.5% lower than diesel.
References
[1] | Huseyin Aydin, Hasan Bayindir, 2010. Performance and emission analysis of cottonseed oil and methyl ester in a diesel engine, Renewable energy,35, 588-592. |
[2] | Md Nurun Nabi, 2009, Biodiesel from cotton seed oil and its effect on engine performance and exhaust emissions, Applied thermal engineering, 29, 2565-2270. |
[3] | Tizane Daho, Gillis Vaiylingom, Salifou, K., Ouiminga et al., 2013, Infuence of engine load and fuel droplet size on performance of a CI engine fueled with cottonseed oil and its blends with diesel fuel, Applied Energy,111, 1046-1053. |
[4] | Misra, R.D., Murthy, M.S., 2011, Performance, emission and combustion evaluation of soapnut oil diesel-diesel blends in a compression ignition engine, Fuel, 90, 2514-2518. |
[5] | Chang Sik Lee, Su Han Park, Hyung jun Kim, HyunKyu Suh, 2009, A study on fuel injection and atomization characteristics of soyabean oil methylester, (SME) international journal of heat and fluid flow, 30, 108-116. |
[6] | Cenk Sayin, Metin Gumus, Mustafa Canakci, 2012, Effect of fuel injection pressure on the injection, combustion and performance characteristics of a DI diesel engine fuelled with canola oil methyl esters-diesel fuel blends”, Biomass and Bioenergy, 46, 435-446. |
[7] | Ismet Çelikten, Atilla Koca, Mehmet Ali Arslan, 2010, Comparison of performance and emissions of diesel fuel, rapeseed and soybean oil methyl esters injected at different pressures, Renewable Energy, 35, 814-820. |
[8] | Avinash Kumar Agarwal, 2007, Performance and emissions characteristics of Jatropha oil (preheated and blends) in a direct injection compression ignition engine, Applied Thermal Engineering, 27, 2314–2323. |
[9] | Godiganur, S., Murthy, C.H.S., Reddy. R, P., 2009, Cummins engine performance and emission tests using methyl ester mahua oil /diesel blends, Renew Energy ,34, 2172-2177. |
[10] | Raheman, H., A, G, Phadatare, A, G., 2004, Diesel engine emissions and performance from blends of karanja methyl ester and diesel, Biomass and Bioenergy, 27, 393 – 397. |
[11] | Jindal, S., B.P Nandawana, B,P., Rathore, N,S, Vashistha, V., 2010, Experiemental investigation of the effect of compression ratio and injection pressure in a diesel engine running on Jatropha methyl ester, Applied thermal engineering, 30, 442-448. |